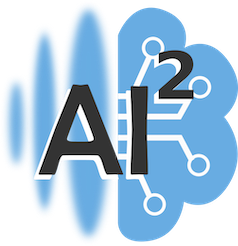
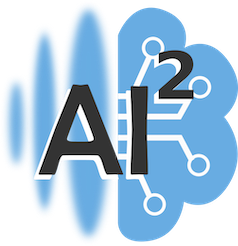
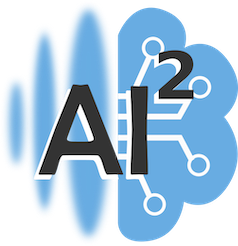
Artificial Intelligence in Atom Interferometers
Algorithms from the field of artificial intelligence (AI) and machine learning have been used in recent years for a variety of applications to efficiently solve multidimensional problems. In physics, these algorithms are applied with increasing success, for example, to solve the Schrödinger equation for many-body problems, or used experimentally to generate ultracold atoms and control lasers. In this project we aim to work on three fundamental pillars of AI in atom interferomtry: theory modelling, measurement data extraction, and operation of experiments.
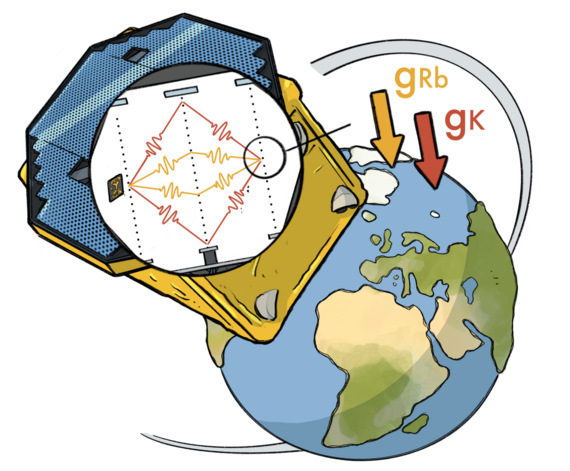
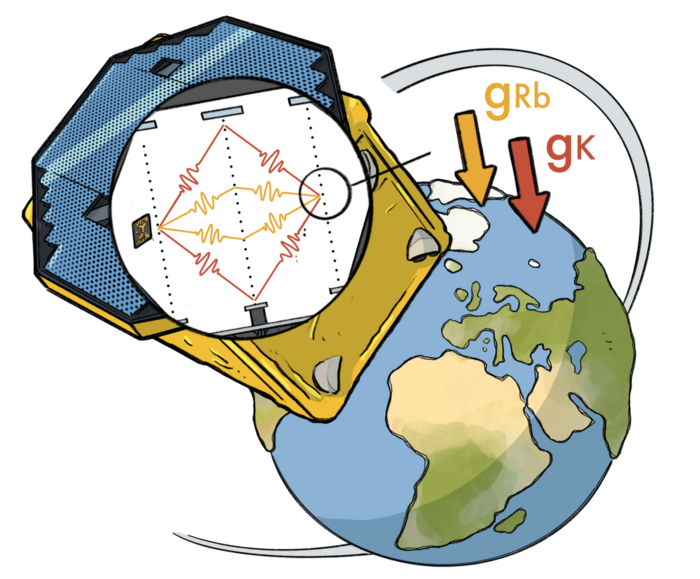
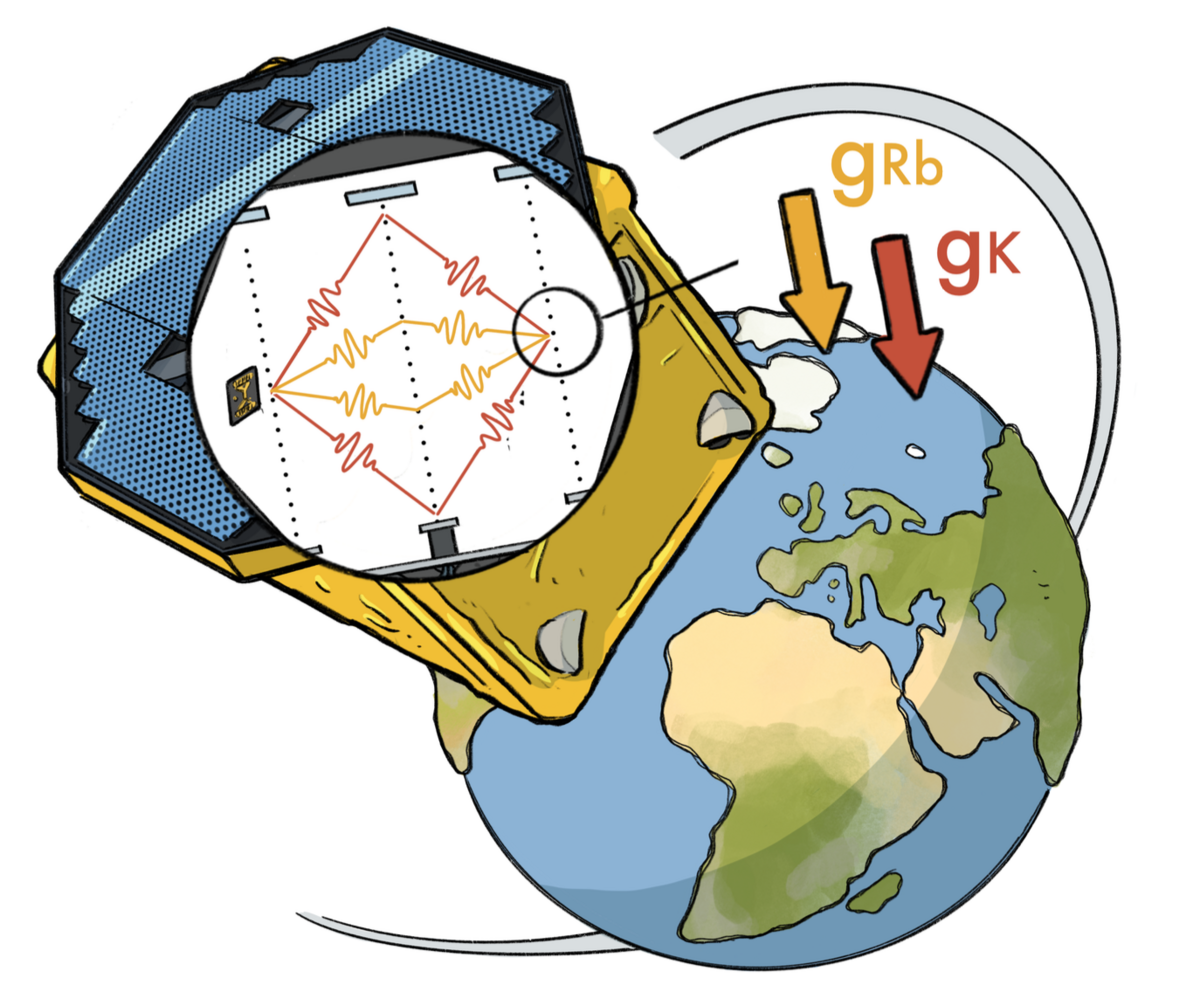
Inertial Sensing in Space
Quantum sensors based on the interference of matter waves provide an exceptional measurement tool for inertial forces, and are considered next generation accelerometers for applications in geodesy, navigation, or fundamental physics due to the absence of drifts. Space-borne atom interferometers promise to exploit the full potential of these sensors due to long free-fall times, and to reach unprecedented sensitivity at low frequencies.
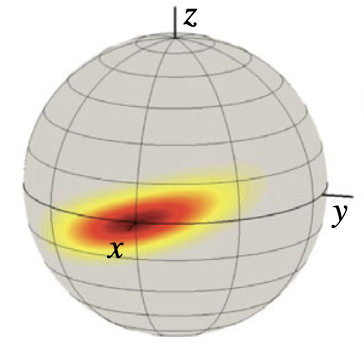
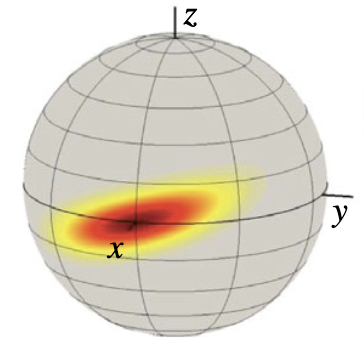
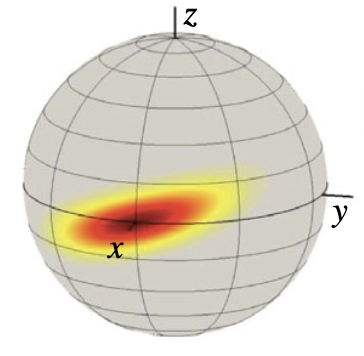
Atom Interferometry with Non-Classical States
Measurements of classical states are inherently sensitivity-limited by the Standard Quantum Limit/ Shot Noise Limit. Utilising non-classical states allows for improved sensitivity beyond this limit and can theoretically approach the lower Heisenberg Limit. One possible method to generate such non-classical states is to reduce the variance of one degree of measurement at the expense of increasing the variance of another one. These processes are referred to as squeezing.
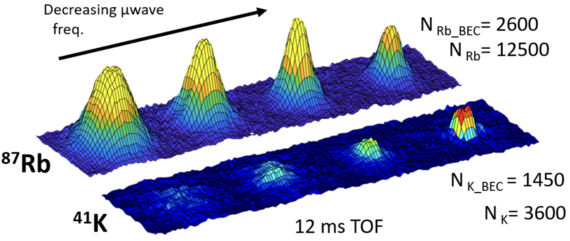
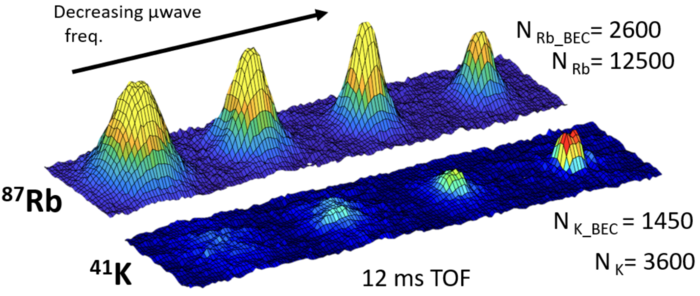
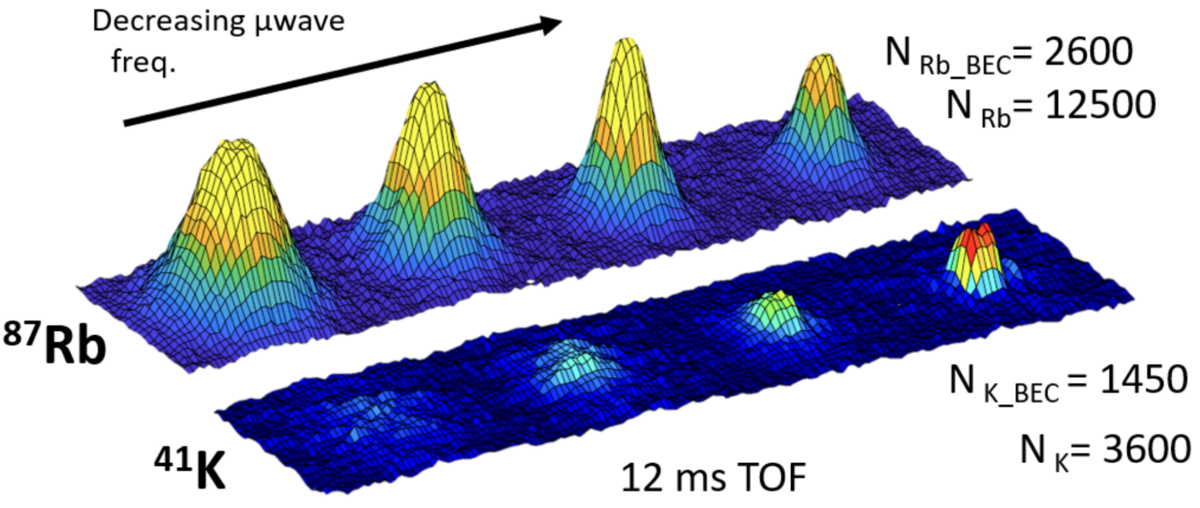
Quantum Gases in Microgravity and Space
Our work focuses on manipulating quantum gases in microgravity, exploring precise control methods and studying quantum mixtures and dual-species Bose-Einstein condensate (BEC) interferometry. Utilizing advanced techniques, we aim to extend free-fall times and conduct experiments in atom interferometry, providing insights into fundamental physics in the absence of gravitational asymmetry. Moreover, the creation of quantum bubbles in microgravity offers a unique platform for studying many-body effects and curved manifolds.
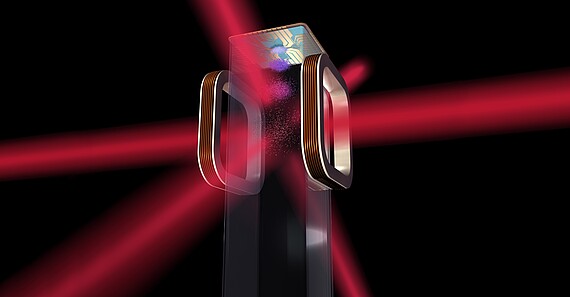
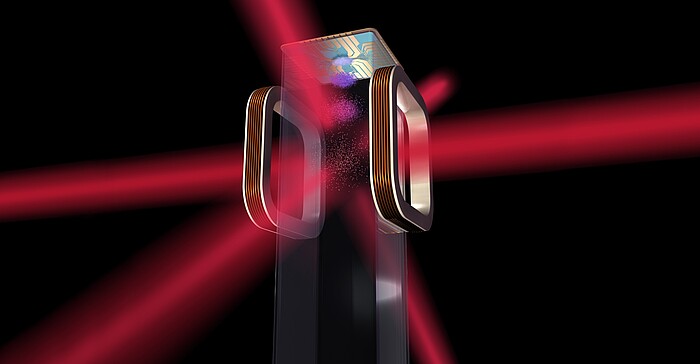
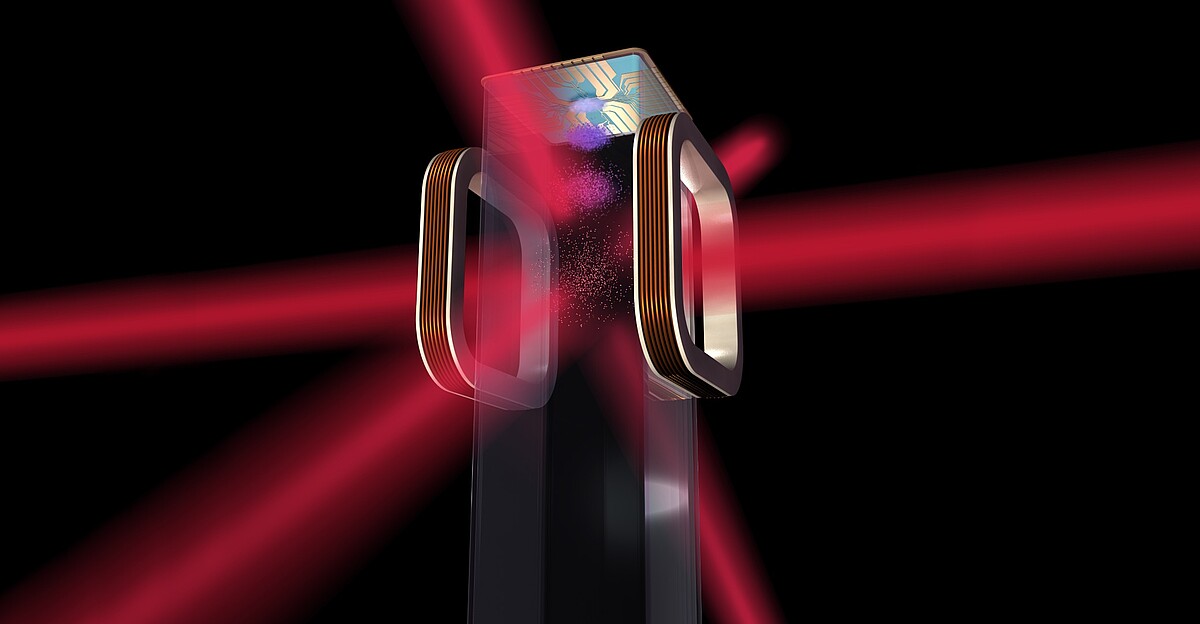
Theory of atom interferometers
Atom interferometry is a successful tool for precision measurements, inertial sensing and search for physics beyond standard model. Today’s experiments provide the state-of-the-art determination of the fine-structure constant [1,2] and the best quantum test of the universality of free fall [3]. Future matter-wave experiments are anticipated to feature extreme momentum or spatial separations as well as interrogation times on the order of seconds. This will enable them to detect signatures of gravitational waves and aid in the search for dark matter and dark energy [4,5]. Ultimately, however, the performance of any sensor is limited by the accuracy of the theoretical models describing its systematic and the statistical errors. Thus, accurate modelling of these sensors is required to leverage their potential to the fullest.
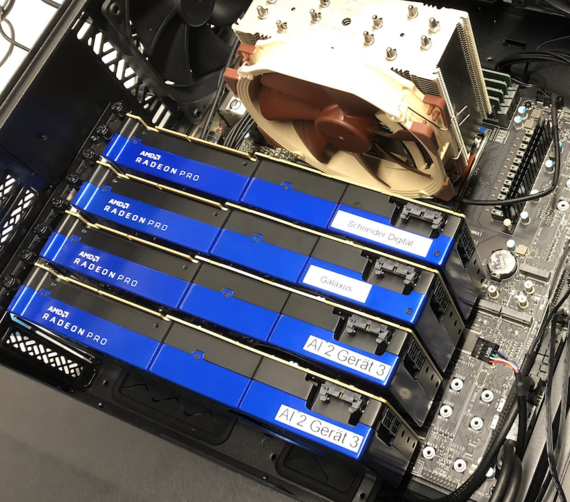
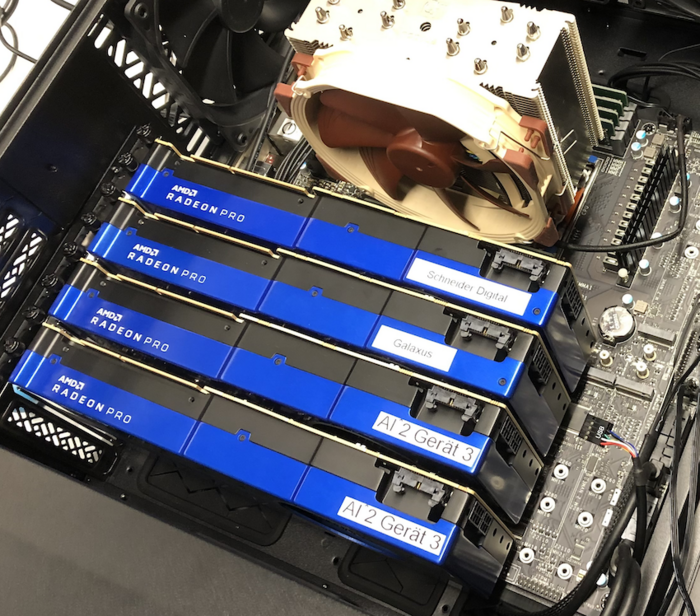
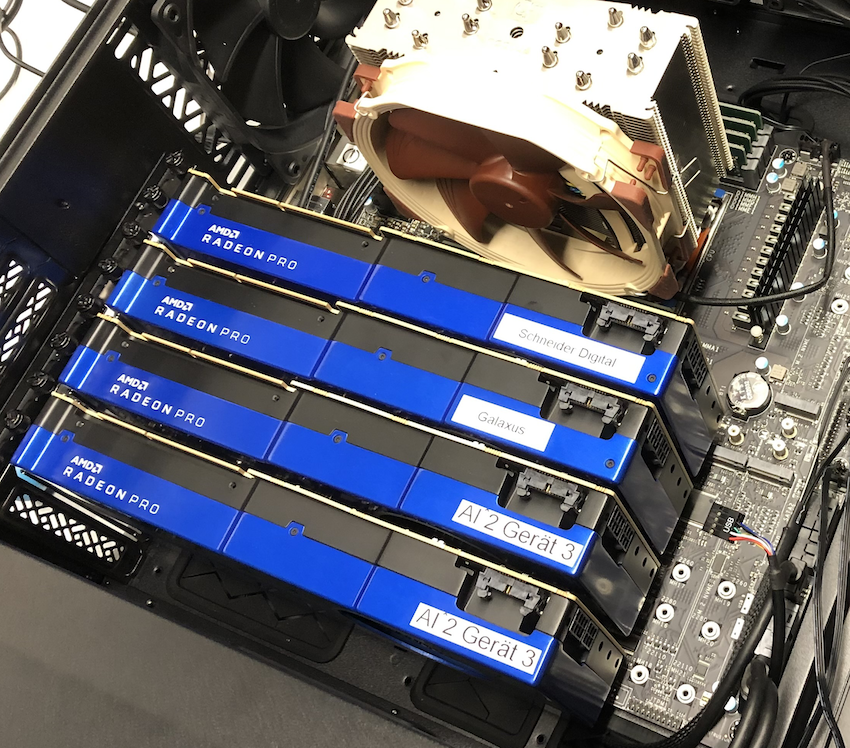
Atom Interferometry Simulation Framework
The position space simulations are a robust approach developed by our group [1] that serves as a foundation for studying complex phenomena in matter wave interferometry. As a fundamental building block for both analytical work and experimental system simulations, it has proven indispensable in advancing our understanding of experiments and fundamental aspects of matter wave interferometry. On this page we provide an overview of the framework and its diverse applications.