Overview
Molecules at ultracold temperatures move at velocities close to a standstill and can be precisely controlled in their quantum state and their orientation in the lab frame. Therefore, their structure and collisional properties can be investigated with much higher precision, enabling fundamental tests of symmetries on the quantum level as well as ultracold chemistry. A dense gas comprised of many ultracold molecules would furthermore represent a system governed by quantum behaviour and long-range interactions allowing the experimentalists to simulate solid state behaviour with unprecedent control and precision, in order to investigate a multitude of phenomena such as superconductivity and new phases of matter. An array of dipolar molecules is furthermore an interesting system for quantum information science, as it would allow for strong, controllable interactions between long-lived qubits.
Our research aims to cool diatomic molecules from 10000K down to ultracold temperatures by the means of buffer gas cooling and direct laser cooling. Calcium monofluoride (CaF) in particular is part of a special class of molecular radicals, having a valence electron which hardly contributes to the molecular bonding. These types of molecules can scatter thousands of laser photons before decaying into higher vibrational states, making them viable candidates for many of the atomic laser cooling schemes employed today. Once slowed, the molecules will be trapped in a magneto-optical trap and transferred to either an optical lattice or an array of tweezers, where we can investigate their scattering and interaction properties.
Cryogenic Buffer Gas Beam Source
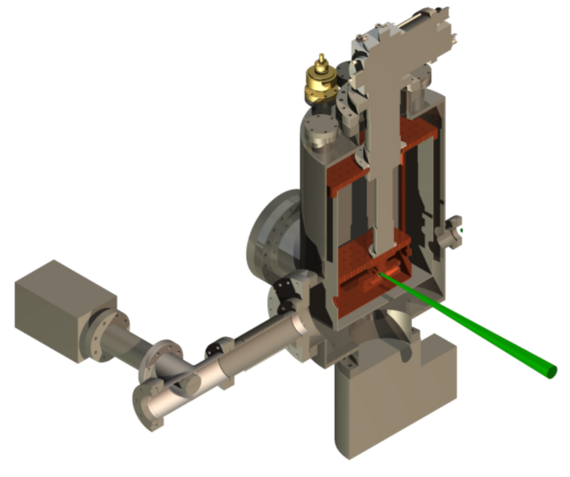
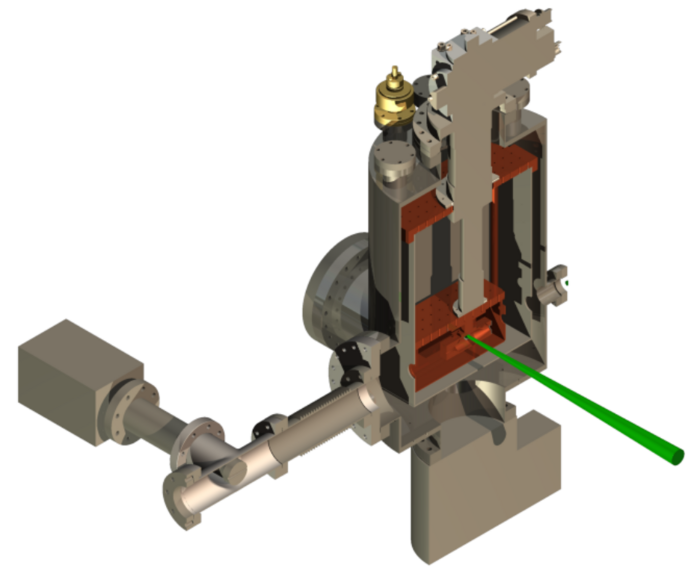
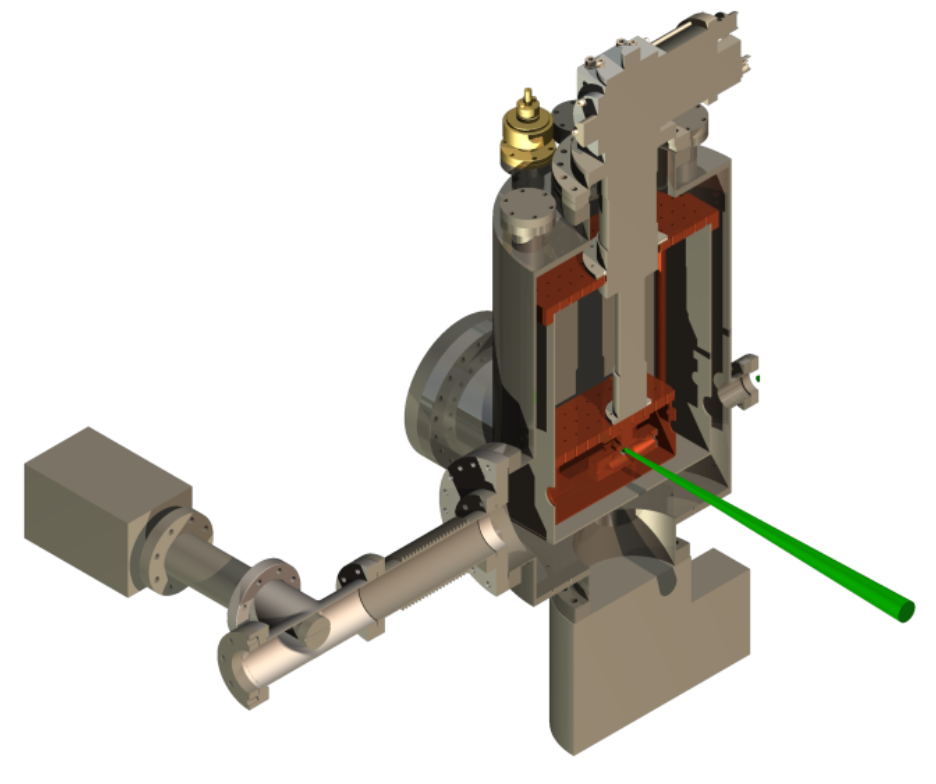
At the start of every experiment in ultracold molecular physics always lies one thing: The molecular source. For us this source comes in the form of a cryogenic buffer gas beam. A calcium metal target is ablated with a high power, pulsed 1064nm laser, ejecting 10000K hot Ca atoms into a small copper cell. Also entering the cell is a stream of 4K cold helium as well as a small amount of SF6 gas. The calcium atoms react with the SF6 to form, amongst other things, CaF molecules, which are then cooled to 4K by the helium background and swept through an opening in the front of the buffer gas cell, entrenched in the helium flow.
Molecular Zeeman Slowing
As the buffer gas beam is too fast (~150m/s) to be trapped in the following stages of the experiment, a slowing method needs to be devised. Many such methods exist, such as white-light slowing, frequency-chirped light slowing, Stark or Zeeman deceleration as well as a molecular centrifuge to name a few. Our group has opted to try out molecular Zeeman slowing, a technique which we proposed should be more efficient than existing methods. It relies on the level structure of CaF in high magnetic fields, where the nuclear spin and rotation of the molecules decouple from their electronic spin, greatly simplifying the energy structure of the ground state. While molecular Zeeman slowing is still a mostly theoretical concept, we have seen first evidence for it working in a laboratory setting.
Magneto-optical Trap
In parallel to investigating the feasibility and efficiency of a molecular Zeeman slower we are setting up a magneto-optical trap (MOT) for our molecules. Standard, red-detuned (two-colour) molecular MOTs are hot and not very dense, owing to very robust sub-Doppler heating effects in these systems. It has however recently been proposed, and even more recently shown, that blue-colour MOTs can be used after initial trapping. These MOTs tend to have sub-Doppler cooling, reducing both the temperature and increasing the density of the molecules. In our group we have proposed several schemes for realizing such a blue-detuned MOT in CaF, either after a red-detuned MOT has been loaded, or even during the loading stage. A continuous way of loading a cold, dense, blue-detuned MOT would enhance molecule numbers and phase space density greatly when combined with the continuous nature of Zeeman slowing, especially as continuous molecular sources are being discovered.
The Team
Principle Investigators


Institut für Quantenoptik
30167 Hannover


Institut für Quantenoptik
30167 Hannover
Institut für Quantenoptik
30167 Hannover
Ph.D. Students
Institut für Quantenoptik
30167 Hannover
Institut für Quantenoptik
Publications
Article
-
(2023): Blue-detuned molecular magneto-optical trap schemes based on bayesian optimization, Physical Review A
DOI: 10.1103/PhysRevA.108.033102
arXiv: arXiv:2305.16576 [physics.atom-ph] -
(2022): Engineering the sub-Doppler force in magneto-optical traps, Phys. Rev. Research 4, L042036 (2022)
DOI: https://doi.org/10.1103/PhysRevResearch.4.L042036
arXiv: arXiv:2203.07754 -
(2021): Characterizing the Zeeman slowing force for 40Ca19F molecules, New Journal of Physics
DOI: https://doi.org/10.1088/1367-2630/ac1ed7
arXiv: 2104.08235 -
(2021): Maximizing the capture velocity of molecular magneto-optical traps with Bayesian optimization, New Journal of Physics
DOI: https://doi.org/10.1088/1367-2630/ac06e6
arXiv: 2104.07984 -
(2018): A Zeeman slower for diatomic molecules, New Journal of Physics, Volume 20, April 2018
DOI: 10.1088/1367-2630/aab9f5 -
(2018): Type-II Zeeman slowing: Characterization and comparison to conventional radiative beam slowing schemes, Phys. Rev. A 98, 063408
DOI: 10.1103/PhysRevA.98.063408
arXiv: arXiv:1811.10404