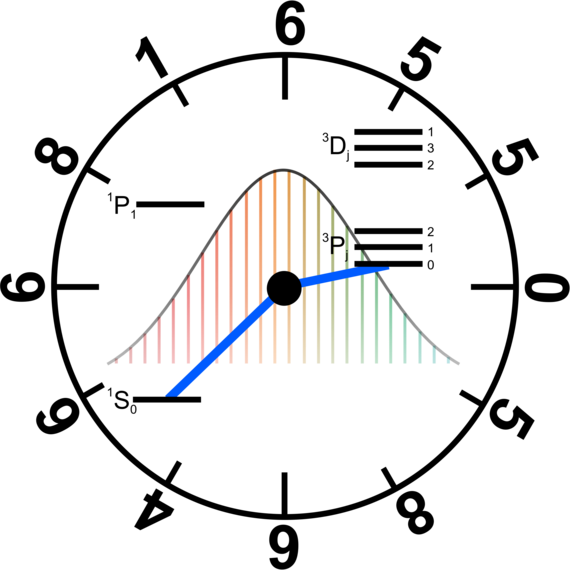
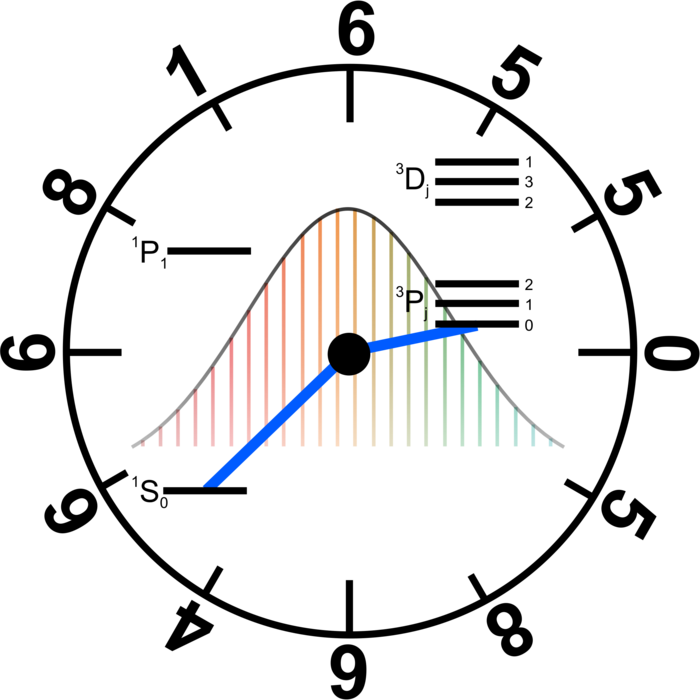
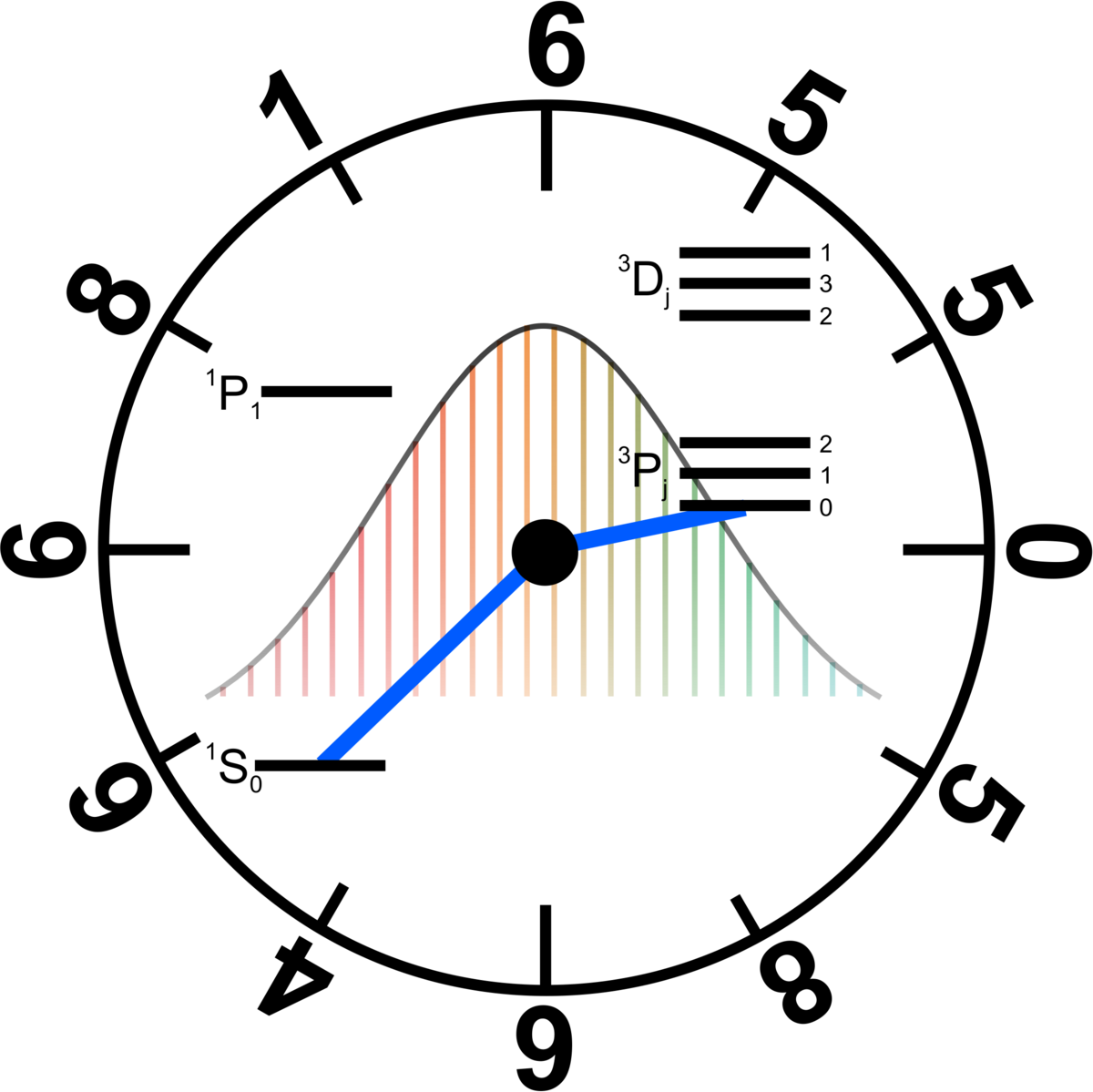
Frequency is the physical quantity today with the highest determined degree of accuracy. Scientists continually aspire to develop more precise frequency standards. Applications of such accurate clocks are in satellite based navigation systems, fiber-optical communication networks, the verification of relativistic effects and many more.
A breakthrough in the modern history of frequency standards was the realization of the method of separated oscillatory fields by Norman F. Ramsey and its application to microwave atomic clocks based on cesium. A giant step forward into the optical domain was paved by the invention of the optical frequency comb (for which J. Hall and T. W. Hänsch were awarded the Nobel prize in 2005), which allows a direct measurement of optical frequencies with very high precision. The first realization of optical atomic clocks was based on the 1So → 3P1 intercombination transition within a Ramsey-Bordé interferometer, where a pre-cooled freefalling atomic sample has been interrogated. With the idea of lattice-based atomic clocks by H. Katori, a Doppler- and recoil-free spectroscopy of the atomic ensemble on the strictly forbidden 1So → 3P0 transition has been demonstrated.
The ongoing optimization by various groups working around the world on optical lattice clocks lead to an uncertainty being less than one second in 13.8 billion years, namely since the big bang! Such an accurate clock is extremely sensitive to the smallest perturbations in the environment, making it a very precise sensor.
A Magnesium Optical Lattice Clock
Our work on an optical frequency standard based on bosonic magnesium is motivated by the quest for precise time and frequency standards, both for technology and fundamental research. In respect to black body radiation (BBR), state-of-the-art lattice clocks with e.g. strontium and ytterbium need a very sophisticated control of the surrounding temperature or even cryogenic environments in order to not be limited by this systematic effect, while magnesium offers an up to one order of magnitude lower sensitivity to BBR. Furthermore, magnesium shows a relatively simple electronic configuration, allowing for highly precise theoretical predictions and simulations.
But on the other hand, cooling and trapping is technically challenging in direct comparison to other lattice clock species, which needed us to develop new methods to prepare cold ensembles of atoms in the optical lattice. With this we were able to demonstrate the first optical lattice clock based on bosonic magnesium with an averaging behaviour of 10-14/√τ which allowed us to reach an instability in the 10-16 regime and perform systematic analysis down to low 10-15. The main limitation was caused by the rather shallow lattice, resulting in a significant tunnel broadening to several hundred hertz and more. After increasing the trap depth we entered the regime of negligible tunnelling, where we observe an almost two orders of magnitude narrower linewidth of a few hertz. With this, we expect an improvement of the averaging behaviour on the same order, which paves the route to systematic evaluation on a much lower level.
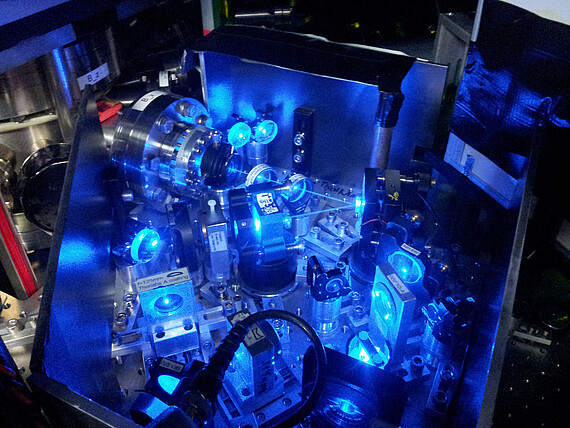
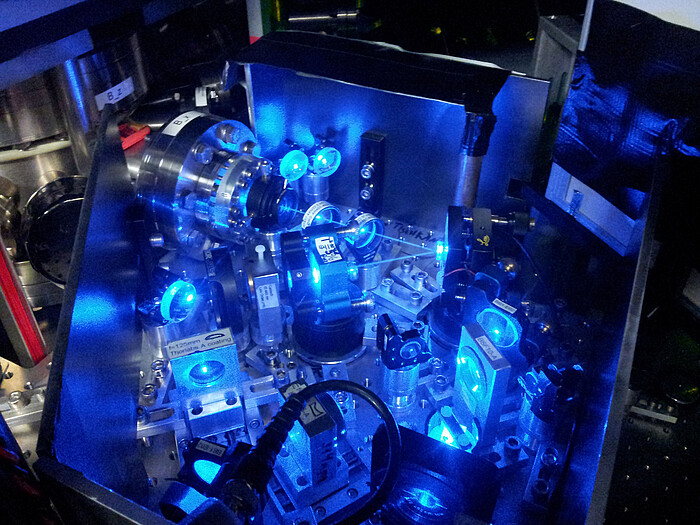
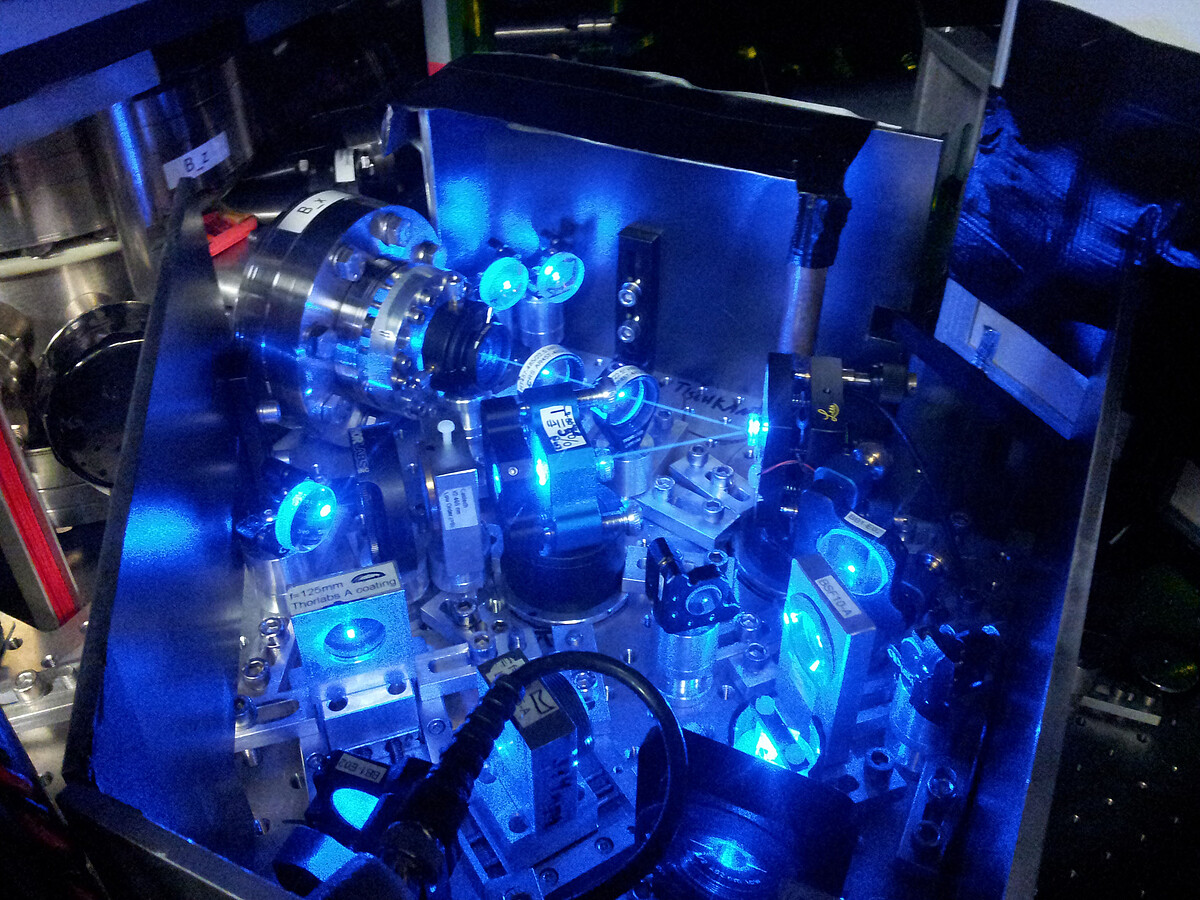
Research Goals
- Absolute frequency measurement in the optical regime
- Optical and radio frequency distribution
- Frequency comparision with other institutes via fiber links
- Exploring new cooling techniques with magnesium
Coordinators


30167 Hannover


Publications
-
(2020): Vertical Gravity Profile in a 10 m Atom Interferometer, J. Geod. 94, 122 (2020)
DOI: 10.1007/s00190-020-01451-y
arXiv: 2003.04875 -
(2015): Towards a Mg Lattice Clock: Observation of the 1S0-3P0 Transition and Determination of the Magic Wavelength, Phys. Rev. Lett. 115, 240801 (2015) Weitere Informationen
DOI: 10.1103/PhysRevLett.115.240801 -
(2012): Beating the density limit by continuously loading a dipole trap from millikelvin-hot magnesium atoms, Phys. Rev. A 86, 043416 (2012) Weitere Informationen
DOI: 10.1103/PhysRevA.86.043416